Main content
Despite encouraging reductions in the global burden of malaria in the early 21st century, progress has since stagnated, particularly in the most heavily affected areas of sub-Saharan Africa. Implementation of an effective malaria vaccine is considered essential to bolster existing tools (e.g., effective case management, intermittent presumptive treatment, seasonal malaria chemoprevention, and vector management) and underpin control, or even perhaps for eradication of malaria. Ideally, a malaria vaccine would be integrated into the World Health Organization’s (WHO) Expanded Programme of Immunisation, benefitting from the programme’s existing management and logistics in order to effectively target those at greatest risk for malaria, i.e. infants and children. So where are we currently in terms of malaria vaccine development do we have a silver bullet in hand, or is it still just a ‘shot’ (of vaccine) in the dark? After presenting some background, we will discuss the current developmental status and prospects of three promising examples of vaccines that target Plasmodium falciparum, the most severe strain of malaria parasites globally.
Rts,s as01 (mosquirixtm)
Development of RTS, S started in the late 1980s, following the discovery that immune responses against P. Falciparum Circumsporozoite Protein (CSP) play an important role in protection against infection. CSP is the most abundant protein on the surface of sporozoites, the infectious form of the parasite transmitted by mosquitoes to people. The RTS, S vaccine consists of recombinant (synthetically produced) sections of CSP, fused and combined with recombinant hepatitis B surface antigen (HBsAg). The addition of HBsAg helps to strengthen the immune response against CSP, but also induces potent immunity against hepatitis B virus. RTS, S is administered in a strong adjuvant, AS01, to further boost immune responses.
Figure I summarises the developmental history of RTS, S, illustrating just how long such processes can take. Initial clinical testing was conducted in trials in malaria-naïve U.S. adult volunteers in the mid-90s, demonstrating safety and protection against Controlled Human Malaria Infections (CHMI). Such studies, in which subjects are deliberately infected with laboratory-cultured malaria parasites under highly controlled conditions, have proven themselves an invaluable tool for advancing the clinical development of promising vaccine candidates. The first trials of RTS, S in African (Gambian) adults were conducted around the millennium, but efficacy against naturally-acquired infection proved somewhat disappointing, protecting against only about 34% of infections. Despite this setback, over the next decade further testing was conducted in children and finally in infants, initially in Mozambique and subsequently in pivotal phase-3 trials in several counties across sub-Saharan Africa. RTS, S has consistently demonstrated a good safety and tolerability profile, but at 18-36% protection against episodes of clinical malaria, efficacy remains well below the target threshold of 75% set by the WHO. [1,2] Protection against severe malaria is lower still (1% to 32%). A partial explanation for this relatively disappointing efficacy against naturally-acquired malaria infection may be that the vaccine only protects well against circulating P. falciparum strains that genetically resemble the vaccine. [3] Moreover, protection is of relatively short duration, waning within 3 years of initial immunisation. Indeed, it has been suggested that RTS, S may even cause a rebound-effect, whereby susceptibility to malaria is increased in the long term compared to unvaccinated subjects, at least in high-transmission areas, due to slower induction of naturally-acquired immunity in vaccinees. [4] In an attempt to overcome waning immunity, a subset of participants in the phase 3 trials were administered a booster dose at 20 months after initial immunisation; over 3-4 years of follow-up, protection was indeed poorer in subjects who did not receive a booster. Importantly, protection is also relatively poorer in areas of high transmission, and in infants as compared to children. [2]
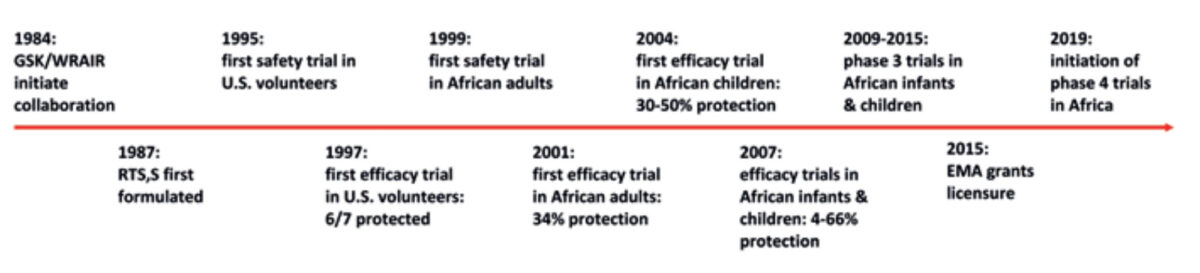
EMA – European Medicines Agency; GSK – GlaxoSmithKline; WRAIR – Walter Reed Army Institute of Research.
Based on these results, in 2015 (some 30 years since its first development) RTS, S nevertheless received regulatory approval from the European Medicines Agency – the first malaria vaccine ever to do so. Before taking a decision on whether to recommend the widescale implementation of RTS, S, however, WHO has requested further large-scale post-licensure trials. These commenced earlier this year in Ghana, Kenya and Malawi and will last until 2024. Some issues that remain to be resolved in these trials are whether it is feasible logistically to target vaccine recipients for a booster dose, and whether the marginally increased (but still extremely low) risk of cerebral malaria and meningitis in children and a slight increase in all-cause mortality in girls as observed in RTS, S recipients in the phase 3 studies represent genuine associations or merely chance post-hoc findings.[5]
Attenuated whole sporozoite-based vaccines
In an attempt to improve upon the protection induced by RTS, S, other researchers have been developing second-generation vaccines consisting of attenuated live sporozoites. Live-attenuated vaccines are widely used against other diseases (e.g., measles, yellow fever and BCG for TBC), are believed to induce stronger, broader and longer-lasting immune responses, and were first pioneered for malaria by Ruth and Victor Nussenzweig in the 1960s and ’70s. [6] Sporozoites can be attenuated by radiation, genetic modification, or concomitant administration of chemoprophylaxis. The objective in all cases is to ensure that these inoculated sporozoites abort their development before themselves becoming pathogenic blood-stage parasites; in the process they are exposed to the immune system, inducing protective immune responses against subsequent infections (Figure 2).
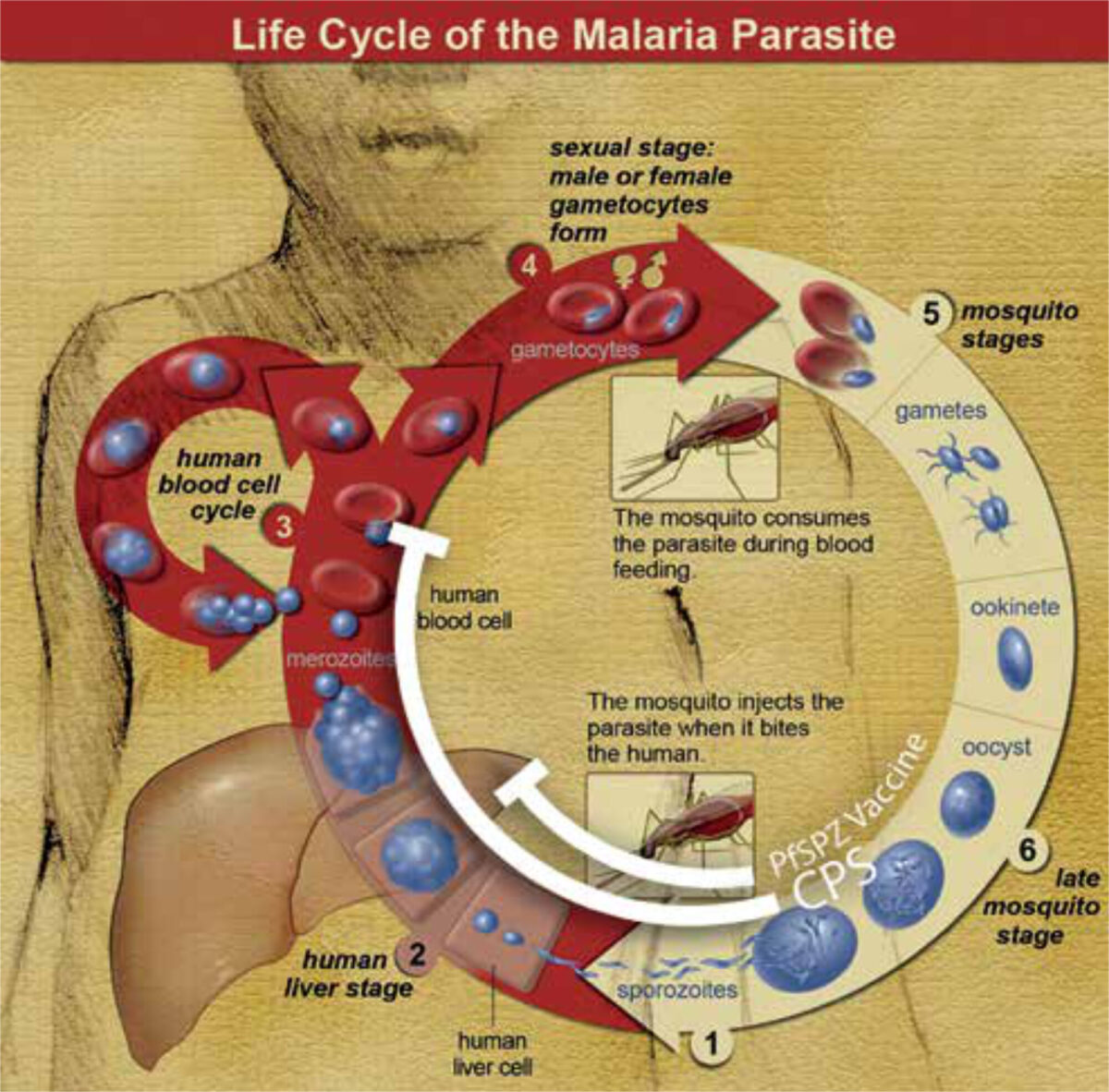
In a landmark study, researchers at Radboud UMC (Nijmegen) pioneered a highly efficacious form of immunisation known as ChemoProphylaxis-with-Sporozoites (CPS), whereby subjects are inoculated with infectious sporozoites by mosquito bites whilst taking anti-malarial prophylaxis (usually chloroquine or mefloquine) to kill any parasites emerging from the liver into the blood-stream. [7] Alongside colleagues from Leiden UMC and Erasmus MC (Rotterdam), they have advanced this concept in a series of successful CHMI studies. This immunisation strategy, although not practically implementable on a large scale in resource-poor settings, remains the most potent known method for inducing immunity against malaria. [8]
The success of CPS has led to resurgent interest in the potential of attenuated whole-sporozoite vaccines. An important player in this field has been the U.S. biotech start-up Sanaria Inc., which pioneered a method to purify live aseptic P. falciparum sporozoites (PfSPZ) from the salivary glands of laboratory-reared mosquitoes and cryopreserve these in vials stored in liquid nitrogen. These can be shipped all over the world, thawed and administered intravenously by needle & syringe. This has helped to accelerate global vaccine development by allowing clinical trials to be conducted where they are most relevant, in resource-poor settings in sub-Saharan Africa. Indeed, Sanaria’s PfSPZ Vaccine, consisting of radiation-attenuated sporozoites, has over the past decade undergone testing in African adults, children and infants in amongst others Tanzania, Kenya, Mali, Gabon and Equatorial Guinee. [9]
Reminiscent of RTS, S however, protection in African populations, particularly infants, appears to be somewhat poorer than in malaria-naïve adult volunteers exposed to CHMI in Europe and the U.S. Potential explanations, including immaturity of young children’s immune systems, immunosuppressive effects of prior malaria exposure and/or helminth co-infections, and parasite strain diversity, remain to be elucidated. Despite these set-backs, PfSPZ Vaccine is set to undergo large-scale testing in a phase 3 trial in Equatorial Guinee later this year.
Transmission-blocking vaccines
Vaccines targeting gametocytes (the parasite forms taken up by mosquitoes to complete malaria’s life cycle) can help to reduce transmission. Although such a vaccine would not directly benefit its recipient, it could reduce cases of malaria in the community and indirectly even the recipient’s own chances of re-infection. Such an altruistic vaccine could conceivably be added to a multi-component vaccine that also induces direct protection against infection or disease.
Several candidate vaccines induce potent transmission-blocking immunity in animal models and are currently undergoing clinical development. A leading example is Pfs48/45, a gametocyte protein shown by researchers at Radboud UMC to form a critical link in mosquito-transmission. A recombinant vaccine, Ro.6C, based on this protein is set to undergo testing here in first-in-human clinical trials in 2020, including assessment of transmission-blocking activity. [10]
Perspectives
After years of largely unsuccessful attempts, exciting progress is now also being made towards a blood-stage malaria vaccine, based on reticulocyte-binding protein homolog 5 (RH5) that plays an essential role in the invasion of erythrocytes by P. falciparum merozoites. A first phase I trial of this vaccine induced antibodies with potent ability to inhibit invasion. [11]
In parallel with the clinical development of these vaccine strategies, state-of-the-art technologies such as genetically-attenuated parasite strains and passive immunisation with recombinant monoclonal antibodies are making inroads in the P. falciparum vaccine field, with significant contributions from researchers in the Netherlands, and offer great promise for the future. Moreover, vaccines against the world’s second most dangerous malaria parasite, P. vivax, are also starting to be developed.
Malaria vaccine development’s slow track record, and particularly the relatively disappointing efficacy of candidate vaccines in naturally-exposed populations, should of course caution against hubris. In parallel with empirical vaccine development, the field needs to develop a better understanding of fundamental obstacles to malaria immunity, including strain-diversity – currently a major area of research focus at amongst others Radboud UMC. That said, rational approaches and dedicated effort have already advanced malaria vaccine development well beyond a proverbial shot in the dark. Several shiny-looking bullets look set to be added to our anti-malarial arsenal and with concerted research one will yet be crafted of true ‘silver’.
References
- Rts S Clinical Trials Partnership. Efficacy and safety of RTS, S/AS01 malaria vaccine with or without a booster dose in infants and children in Africa: final results of a phase 3, individually randomised, controlled trial. Lancet. 2015;386(9988):31-45.
- Moorthy VS, Newman RD, Okwo-Bele J-M. Malaria vaccine technology roadmap. Lancet. 2013;382(9906):1700-1.
- Neafsey DE, Juraska M, Bedford T et al. Genetic diversity and protective efficacy of the RTS,S/ASOI malaria vaccine. N Engl J Med. 2015;373(21):2025-37.
- Olotu A, Fegan G, Wambua J et al. Seven-Year Efficacy of RTS, S/AS01 malaria vaccine among young African children. N Engl J Med. 2016;374(26):2519-29.
- Guerra Mendoza Y, Garric E et al. Safety profile of the RTS,S/AS01 malaria vaccine in infants and children: additional data from a phase III randomized controlled trial in sub-Saharan Africa. Hum Vaccin Immunother. 2019;15(10):2386-98.
- Nussenzweig RS, Vanderberg J, Most H et al. Protective immunity produced by the injection of x-irradiated sporozoites of plasmodium berghei. Nature. 1967;216 (5111):160-2.
- Roestenberg M, McCall M, Hopman J et al. Protection against a malaria challenge by sporozoite inoculation. N Engl J Med. 2009;361(5):468-77.
- Sauerwein RW, Bijker EM, Richie TL. Empowering malaria vaccination by drug administration. Curr Opin Immunol. 2010;22(3):367-73.
- Richie TL, Billingsley PF, Sim BKL et al. Progress with Plasmodium falciparum sporozoite (PfSPZ)-based malaria vaccines. Vaccine. 2015;33(52):7452-61.
- Singh SK, Roeffen W, Andersen G et al. A Plasmodium falciparum 48/45 single epitope Ro.6C subunit protein elicits high levels of transmission blocking antibodies. Vaccine. 2015;33(16):1981-6.
- Payne RO, Silk SE, Elias SC et al. Human vaccination against RH5 induces neutralizing antimalarial antibodies that inhibit RH5 invasion complex interactions. JCI Insight. 2017;2(21).