Main content
Tuberculosis (TB) continues to pose an enormous threat to global health, with over one quarter of the world’s population latently infected, over 10 million new active TB cases each year, over 1.3 million annual deaths, and an ever-rising frequency of multi-resistant Mtb strains. [1] Classical intradermal M.bovis Bacillus Calmette-Guerin (BCG) vaccination of neonates and young infants protects against severe forms of acute and early TB disease, with over 80% protective efficacy against TB meningitis, which can be rapidly fatal. Unfortunately, however, BCG vaccination fails to protect consistently and sufficiently against pulmonary TB in adults, which is the main form of contagious TB. [2] The reasons for this deficiency are not fully understood but may involve waning of memory, interference by other infections such as Non Tuberculous Mycobacterial (NTM) or certain viral infections (CMV, EBV) which can modulate immune responses. As a result BCG vaccination has relatively little impact on global TB transmission patterns, which is a reason for TB vaccine researchers and developers to promote TB vaccines that target adolescent TB.
Developing new tb vaccines
Better TB vaccines could have significant impact against TB, and pre-and post-exposure vaccination with improved vaccines represents a cornerstone of the WHO End TB strategy which aims to end TB by 2035.[1] To discover and develop better TB vaccines, research efforts were initiated in the mid-1990s, sponsored both by private and public funding, particularly the European Commission. [3] Discovery and evaluation of new candidate TB vaccines initially focused on the preclinical and early clinical space, from which emerged a first candidate, namely MVA85A. This vaccine was based on a single Mycobacterium tuberculosis (Mtb) protein, called Ag85A, which was expressed in a non-replicating viral vector (MVA) and had been designed as a booster vaccine for individuals that already have been given BCG. Although the vaccine was immunogenic and protective in certain animal models of TB, it was unable to provide any additional protective efficacy against TB when administered as a booster vaccine following BCG, in a large phase 2b study in infants. [4] This disappointing result, which could have been due to the fact that the Ag85A antigen is insufficiently expressed by Mtb bacteria in the lung, [5] spurred additional efforts to develop better vaccines.
Using diverse approaches enhances chance of success
Five approaches have been actively pursued in the last decade:[3]
- Subunit vaccines which are based on specific immunogenic components of the bacillus, such as proteins, fusion proteins and lipids, which are typically admixed with innate immunity stimulating adjuvants;
- Virally vectored subunit vaccines in which the subunit component is typically expressed from a genetic insert encoding a selected Mtb antigen (such as was the case for MVA85A);
- Improving BCG, by inserting additional antigens or by genetic manipulation of the BCG genome to improve its immunogenicity;
- Attenuating Mtb by deleting essential virulence genes, thus producing a safe and antigenically fully competent Mtb-like vaccine;
- Alternative delivery routes using existing vaccines, such as BCG, for example via the mucosal route (the lung) assuming that the natural route of infection is the most relevant route of vaccine delivery, inducing local immunity and immune (resident) memory.
Recent successes in tb vaccine development
Candidate vaccines from all five categories are being evaluated for safety and immunogenicity in clinical phase 1/2 studies, and some already have been or are being evaluated in phase 2B/3 studies for vaccine efficacy (VE) using prevention of disease (PoD) or prevention of infection (PoI) as primary endpoints (Figure 1).
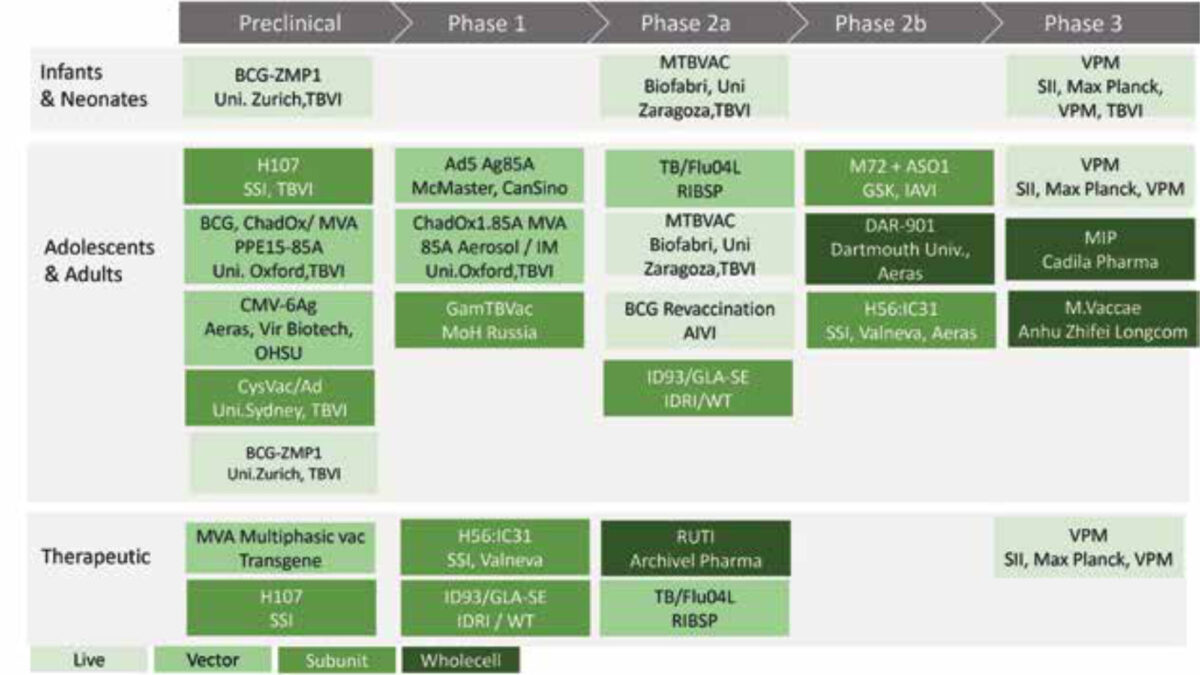
Recently the results from two large studies were reported, with highly encouraging outcomes, strongly suggesting that better TB vaccines indeed are possible. The most important results came from a study in which a subunit vaccine (category 1 above), called M72 (a fusion protein consisting of 2 immunogenic Mtb antigens), was given together with a strong adjuvant, called ASOIE, to adults who were already latently (that is asymptomatically) infected with Mtb. [6] Most Mtb infected people who will develop TB in their lifetime will do so in the first two years after infection. The final 3-year follow-up report was recently reported. Encouragingly, the vaccine arm showed a 50% vaccine efficacy against developing TB, although a relatively limited number of cases was present in the control arm of this large phase 2B trial. Nevertheless, M72 has significant PoD VE, in the absence of major adverse effects.
This encouraging result needs follow up for longer periods of time in order to determine the longevity of the response, and to assess whether further boosting would be required to maintain or augment the VE. At this stage, the mechanism of action of M72+AS01E is unknown. It could involve innate immune driven responses, including trained innate immune memory of myeloid cells, and/or adaptive immune responses (clear CD4+ T cell responses were induced by M72) or both. [3,7] Understanding the immune mechanisms behind the protective effect will be important in order to systematically improve and further develop this or other similar vaccines. Besides determining the longevity of the protective effect, another relevant question is whether M72 also works well in non-Mtb infected persons, including in BCG vaccinated individuals. Notwithstanding these questions, this landmark M72 trial result represents the first promising signal for any new TB vaccine since a century, and now needs to be evaluated in large phase 3 trials in both infected and uninfected people.
A second promising result came from a BCG revaccination study. [8] In this complex study design both a subunit (H4/IC31) and a BCG revaccination arm were included in a setting in which (sustained) PoI rather than PoD was assessed. Infection was defined as having developed a positive immune test (IGRA test) against Mtb specific antigens. The most important result, which had not been anticipated, was that although neither vaccine protected against infection (i.e. IGRA conversion from negative to positive) only BCG revaccination protected significantly against sustained Mtb infection: in the BCG revaccination arm fewer persons had remained IGRA positive after 24 months, representing a 45% VE signal. This finding could suggest that the immune system is able to eradicate an already established infection from the human body once properly activated, in this case by revaccination. This interesting and important concept needs to be examined further, including novel animal models, as it could focus research efforts on Mtb eradication by vaccination.
Understanding the immune mechanisms behind the protective effect
New clinical and promising late preclinical approaches
There are, as already outlined in Figure 1, several candidate vaccines in early stage clinical development. This includes live vaccines such as recombinant BCG and attenuated MTBVAC, other subunit vaccines such as H56, and aerosol based delivery of BCG into the human lung. [7] In parallel, advanced preclinical evaluation models (usually in non-human primates (NHP)) are being used to assess and compare additional TB vaccine delivery routes and systems. Some promising ones include: aerosol / pulmonary delivery of BCG in NHP, with excellent VE results; [9] intravenous delivery of BCG (with strong VE results; Seder et al, unpublished), and Rhesus monkey-CMV vectored multi-antigen subunit vaccines that are being tested in NHP models, again with striking VE effects. [10] In addition, combinatorial vaccines with heterologous prime/boost-regimens can likely be harnessed to further optimize protective immunity induced by vaccination.
The importance of tb correlates of protection
‘Correlates’, often referred to as ‘biomarkers’, are markers that correlate with important biological or medical outcomes, for example disease or protection. Unfortunately, there are virtually no human correlates of protection against TB. This is a major bottleneck delaying TB vaccine evaluation and prioritisation, because such correlates could help to identify protective antigens, develop improved vaccines, and allow the demonstration of immunogenicity and potential VE at an early stage. Correlates would thus facilitate the selection and prioritisation of candidate TB vaccines for human clinical efficacy testing, and help reduce the protracted time scale, large size, and expense of human efficacy trials, thus significantly facilitating TB vaccine development. In addition, correlates could help guide preclinical animal studies and thus help minimize use of animals. Samples from well-defined human cohorts with various Mtb exposure or infection states, including long-term resisters (either resisting natural infection induced IGRA conversion or resisting TB progression once infected), samples from future controlled human mycobacterial challenge models, and – particularly important from individuals from trials with TB vaccines demonstrating protective VE will be essential for accelerating correlate discovery, testing and validation.
In addition, correlates of risk for progressing from asymptomatic (latent) infection towards TB disease would be extremely useful, e.g. in stratifying individuals in observational and clinical intervention studies, including (therapeutic) vaccination and drug studies. Several first signatures were reported a few years ago [11-13], and some of these are currently being further refined (e.g. [14,15]).
Although beyond the scope of this short review, it is clear that correlate discovery and evaluation is a second major priority in the field of TB vaccine development.
Concluding future outlook
In very recent years, TB vaccine research and TB correlate discovery have witnessed significant breakthroughs. This provides real hope for effective, life-saving TB vaccines, which are much needed to control the TB endemic, including multi-drug-resistant and extensively drug resistant TB, and to help reach the End TB goal by 2035.[1]
References
- WHO. Global Tuberculosis Report. 2019.
- Ottenhoff TH, Kaufmann SH. Vaccines against tuberculosis: where are we and where do we need to go? PLoS pathogens. 2012;8(5):21002607.
- Kaufmann SHE, Dockrell HM, Drager N et al. TBVAC2020: Advancing Tuberculosis Vaccines from Discovery to Clinical Development. Frontiers in immunology. 2017;8:1203.
- Tameris MD, Hatherill M, Landry BS et al. Safety and efficacy of MVA85A, a new tuberculosis vaccine, in infants previously vaccinated with BCG: a randomised, placebo-controlled phase 2b trial. Lancet 2013;381(9871):1021-8.
- Coppola M, van Meijgaarden KE, Franken KL et al. New Genome-Wide Algorithm Identifies Novel In-Vivo Expressed Mycobacterium Tuberculosis Antigens Inducing Human T-Cell Responses with Classical and Unconventional Cytokine Profiles. Scientific reports. 2016;6:37793.
- Tait DR, Hatherill M, Van Der Meeren O et al. Final analysis of a trial of M72/AS01E vaccine to prevent Tuberculosis. New England J Med, 29th October 2019.
- Andersen P, Scriba TJ. Moving tuberculosis vaccines from theory to practice. Nature Reviews Immunology. 2019;19(9):550-62.
- Nemes E, Geldenhuys H, Rozot V et al. Prevention of M. tuberculosis Infection with H4:IC31 Vaccine or BCG Revaccination. The New England journal of medicine. 2018;379(2):138-49.
- Dijkman K, Sombroek CC, Vervenne RAW et al. Prevention of tuberculosis infection and disease by local BCG in repeatedly exposed rhesus macaques. Nature Medicine. 2019;25(2):255-62.
- Hansen SG, Zak DE, Xu G et al. Prevention of tuberculosis in rhesus macaques by a cytomegalovirus-based vaccine. Nature Medicine. 2018;24(2):130-43.
- Zak DE, Penn-Nicholson A, Scriba TJ et al. A blood RNA signature for tuberculosis disease risk: a prospective cohort study. Lancet 2016;387(10035):2312-22.
- Fletcher HA, Snowden MA, Landry B et al. T-cell activation is an immune correlate of risk in BCG vaccinated infants. Nature Communications. 2016;7:11290.
- Sloot R. Schim van der Loeff MF, van Zwet EW et al. Biomarkers can identify pulmonary Tuberculosis in HIV-infected drug users months prior to clinical diagnosis. EBioMedicine. 2015;2(2):172-9.
- Suliman S, Luabeya AKK, Geldenhuys H et al. Dose Optimization of H56:IC31 Vaccine for Tuberculosis-endemic populations. A double-blind, placebo-controlled, dose-selection trial. American J Respirat Critical Care Medicine. 2019;199(2):220-31.
- Penn-Nicholson A, Hraha T, Thompson EG et al. Correction: Discovery and validation of a prognostic proteomic signature for tuberculosis progression: A prospective cohort study. PLoS Medicine. 2019;16(7):21002880.